Introduction
Secondary, or rechargeable, lithium-ion batteries are powering tomorrow’s technology, which means the demand for high performance is more critical than ever. Battery packs for electric vehicles and energy storage are required to last longer, charge faster, and hold more energy. A key component of these performance improvements is the efficiency of the battery. Internal defects and aging can cause batteries to not fully charge, not fully discharge, or overheat under load. This is wasted energy for the user. Therefore, it’s important for manufacturers and battery researchers to measure values like DC internal resistance (DCIR) to identify low-quality batteries or to help study degradation behaviors. Source measure units (SMUs), like Keithley’s 24xx Series Graphical Touchscreen SMUs with Test Script Processor (TSP) capability, can make measuring a parameter like DCIR simple. This application note describes how to use the DCIR TSP App for the 24xx Series SMUs to measure the internal resistance of a battery cell.
What is Battery Internal Resistance?
An ideal battery has no internal resistance. Internal resistance in a battery comes from any part of the battery that could inhibit current flow. This includes poor connections between the electrolyte and the electrodes, poor welds between the electrodes and the tabs, or other imperfections. These imperfections cause the internal resistance of the battery to increase. The internal resistance can also change as the battery degrades, which causes the chemical reaction to behave differently, making battery internal resistance a useful way to study life span and performance.
When the battery is charging or discharging, any resistance in the circuit dissipates some energy as heat, which is pure loss from the system. Batteries with higher internal resistance are less efficient, lower performing, and can lead to larger failures due to overheating and thermal runaway. Therefore, measuring internal resistance is a key indicator of the quality of the battery during manufacturing. A high-quality battery will have an internal resistance in the mΩ ranges.
Another reason internal resistance is a useful measurement to make is that batteries are complex, and we must break them down into models of simpler components. This makes modeling and studying battery behavior in circuits easier. The simplest model of a battery can be broken into two pieces: an ideal voltage source that outputs the battery open circuit voltage (OCV) and a series internal resistance (Rin) as shown in Figure 1. Measuring the battery resistance and the open circuit voltage can give insights into the state of charge of the battery.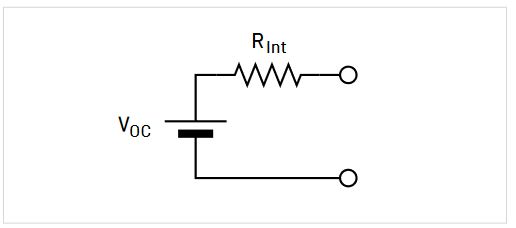
AC methods such as electrochemical impedance spectroscopy (EIS) are popular for research, as this measures capacitive behaviors in the battery. Another method, AC internal resistance (ACIR) can determine internal resistance of a battery; however, this method only looks at ohmic resistance, and does not include resistance from other sources. DCIR includes some resistance due to chemical behaviors. For the purposes of this application note, we will focus on DCIR method only.
Measuring Battery DCIR
The measurement procedure for DCIR involves taking a measurement on the battery under load conditions. For rechargeable batteries, a similar process can be done with a charging current. The challenge with this is that the battery will be discharging (or charging depending on the current direction), which will change the state of charge of the battery and therefore the resistance. The solution for this is to use pulses so that the battery is discharged for a short period of time and then measured.
The most basic procedure includes two steps: the initial baseline measurement and a second measurement after the battery has been loaded. The first measurement can be taken as the open circuit voltage, or it can be taken after a smaller discharge pulse. The measurement procedure is shown in Figure 2.

The value for the internal resistance is then calculated as follows:
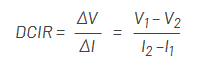
where V1 and I1 are the measured voltage and load current before the pulse or at the end of the first pulse, and V2 and I2 are the measured voltage and load current after the final pulse. If the initial measurement is taken while the battery is at rest, the equation becomes:

The load current used depends on the capacity of the battery and the desired discharge time. These parameters may be defined in testing standards such as IEC 61960. This procedure is outlined in Figure 3.
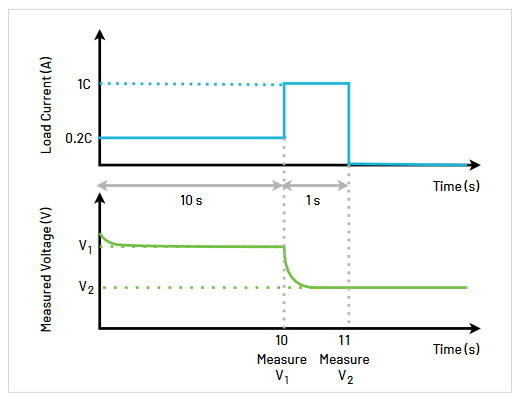
DCIR With a Keithley SMU and TSP Technology
Internal resistance measurements require the instrument to accurately measure the voltage of the battery while acting as a load or a source. The best instrument for this task is a source measure unit or SMU. The Keithley 24xx Series Graphical Touchscreen SMUs are a good option for DCIR measurements because they can act as either a current sink or a current source for rechargeable batteries, while taking measurements. Since the pulses may be done at current rates larger than 1C, the 2460 and 2461 High Current SMUs are the best solution, offering up to 7 A DC current sourcing. The 24xx Series SMUs are all programmable using TSP technology, a custom programming language for instrument automation. The SMU can be controlled from the PC using TSP commands, but it can also host scripts and apps itself. TSP apps such as the DCIR App leverage the large touchscreen front panel to make collecting specific measurements easier.
Before making a DCIR measurement, we must ensure that the SMU is set to high impedance output mode so that the battery is not discharged by the SMU while idle. The DCIR App does this automatically when opened. Once the SMU is in a high impedance state, the battery can be connected as shown in Figure 4. Four wire connections are necessary to eliminate errors due to lead resistance, since battery internal resistance is typically less than 1Ω.
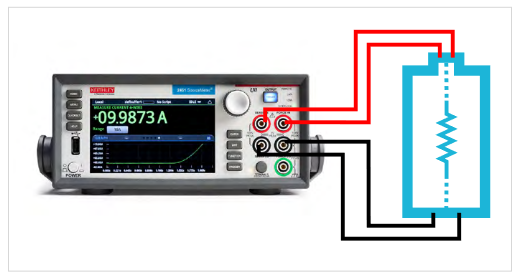
Using the DCIR TSP App
For the purposes of this demo, the app was run with a 2461 High Current SMU and an Ultralast 3400 mAh 18650 LithiumIon battery cell. The battery should be disconnected from the SMU before running the app to minimize discharge.
The app can be downloaded from tek.com and loaded onto a USB drive. Insert the USB drive into the SMU and navigate to the Apps screen by pressing the MENU button and selecting Apps. The app will appear in the USB tab and can be run directly from the USB or saved into the SMU’s memory. Selecting the app icon displays the app information and the Run button as shown in Figure 5.

The app begins by resetting the SMU and setting the off state to high impedance. A popup displays as shown in Figure 6 when this is complete, and the battery can be connected.
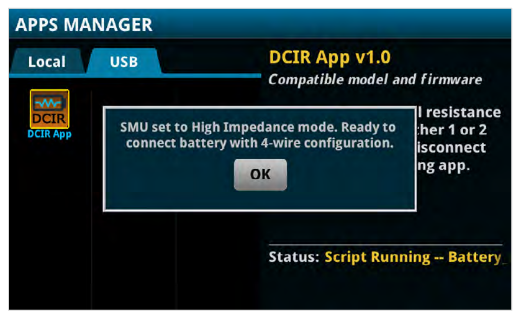
Press OK on the popup to clear it, and proceed to the main app screen as shown in Figure 7.

The voltage limit prevents the SMU from applying a voltage that could damage the battery. This value should be set slightly larger than the maximum voltage of the battery. The default setting is 25% over a nominal 3.6 V battery or 4.5 V. In the center of the app screen are the settings for each pulse. The first pulse can be removed, and a single OCV measurement can be used by selecting the Choose OCV check box. Each pulse can be entered in terms of a current value or as a C Rate and capacity in mAh. Pulses are assumed to be discharging (negative current) unless specified by the Charging Pulse checkbox. When using charging pulses, a pop up will appear to confirm the settings, as applying charge pulses can damage a nonrechargeable battery. Scrolling down the screen displays the timing for each pulse.
For this demo, we will be following the IEC 61690 standard for our 3400 mAh battery. Therefore, we’ve entered a C Rate of 0.2 C for 10 s for pulse 1 and a C Rate of 1 C for 1 s for pulse 2. Both of these pulses are discharging pulses. The app setup is shown in Figure 8.
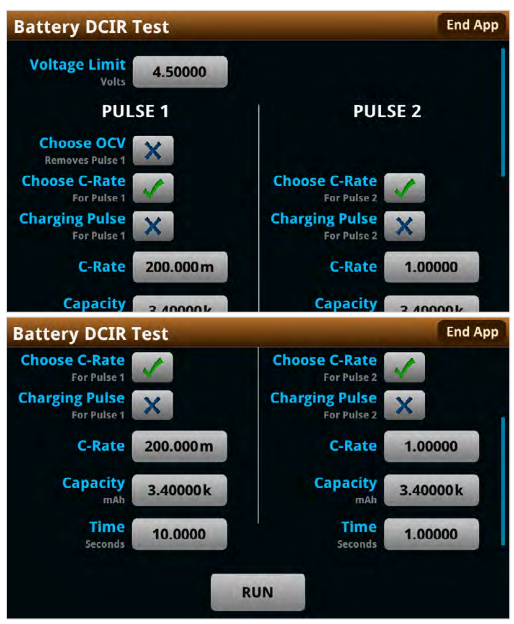
Touch the RUN button below the pulse settings to run the test. The SMU output will turn on and then populate the measurement in the table at the bottom of the screen as shown in Figure 9.

The OCV of the battery is always taken as part of the measurements, but it is only used in the IR calculation if the "Choose OCV" check box is selected.
The app can be rerun as many times as necessary and the data will be appended to a customer buffer (dcirBuffer). This custom buffer can be formatted and saved to a USB drive using the Save Data button. To view the buffer on the unit or clear it, press the MENU button on the front panel, which will display the App Menu screen as shown in Figure 10. The test settings can be accessed again from this same menu.
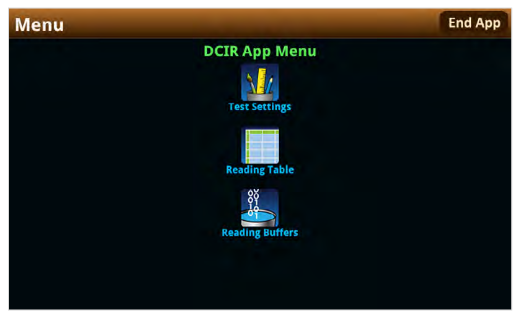
Conclusion
DCIR for a battery has many uses, from helping to model battery behavior and degradation mechanisms to identifying defective batteries. Source measure units like the Keithley 24xx Series Graphical Touchscreen SMUs provide the functionality to run this test with a single instrument. Leveraging the TSP scripting functionality and user-friendly GUI on these instruments brings a new level of convenience for researchers and manufacturers looking to ensure the highest quality in their batteries.
Find more valuable resources at TEK.COM
Copyright © Tektronix. All rights reserved. Tektronix products are covered by U.S. and foreign patents, issued and pending. Information in this publication supersedes that in all previously published material. Specification and price change privileges reserved. TEKTRONIX and TEK are registered trademarks of Tektronix, Inc. All other trade names referenced are the service marks, trademarks or registered trademarks of their respective companies.
050423 1KW-74003-0